Scattering and Diffraction
X-ray Diffraction and Scattering is the most widely used category of synchrotron methods, especially for geosciences. These methods rely on the wavelength of the X-rays, which are comparable to the spacing between atoms in solids, crystals, and even most liquids. When light of any wavelength hits a material, it can scatter, changing the direction of travel. For high-energy X-rays, this scattering is very unlikely except when the wavelength, λ, of the X-ray, precisely matches the spacing between planes of atoms in a crystal. This X-ray diffraction gives strong X-ray scattering intensity at particular scattering angles that depend on the spacing between atoms in the crystal and their orientation to the incident X-rays. From the patterns of X-ray scattering, often while spinning the crystal in the X-ray beam, the details of the atomic structure of the crystal can be worked out. X-ray diffraction can be measured either from intensities of X-rays scattered through a sample, called Laue diffraction, or reflected from the sample, called Bragg diffraction.
For many decades, geoscientists have used laboratory X-ray diffraction to determine the crystal structures of minerals, which is vital to our understanding of the composition of the Earth. Synchrotron X-ray beamlines such as those run by SEES allow much faster and more detailed structural analysis on ever smaller minerals and at extreme conditions of temperature and pressure. The basic X-ray diffraction methods can also be extended away from determining the bulk crystal structure of a mineral to give accurate measures of strain and dislocations in crystals, how phase transitions and melting occur, and details about how the surfaces of crystals relax away from their bulk structure.
Surface X-ray Diffraction
X-ray diffraction of a large single crystal gives intensities at very sharp points (Bragg points) in scattering angle, as there can be millions of planes of atoms participating in the coherent scattering and a slight deviation in angle would put the X-rays scattered from different planes slightly out of phase with one another. However, at the surface of the crystal, the repeated sequence of planes of atoms ends, and so does the scattering of X-rays. The surface therefore affects the X-ray scattering away from the Bragg points, smearing scattered intensities along the lines that connect the Bragg points, and with scattered intensity that falls off dramatically with the angular distance from the Bragg points. These crystal truncation rods depend on the structure of the atoms at the surface of the crystal – typically within a few atomic layers or nanometers from the surface.
Geochemically, the surfaces of minerals are often not simply the bulk crystal terminating perfectly. Instead, the surfaces can relax in structure or even rearrange the top-most layers of atoms, and react with the atmosphere, water, impurities, or contaminants. Using surface-enhanced X-ray diffraction methods like crystal-truncation-rod scattering allows geologists to determine the atomic-scale surface relaxation on minerals, the reactivity of these surfaces, and how contaminants and aqueous ions will interact with them.
Laser-Heated Diamond-Anvil Cell X-ray Diffraction
Diamonds are the strongest material known, perfect crystals, and are nearly transparent to both high-energy X-rays and visible light. Because of these properties, they can be used to recreate the extremely high pressures of the center of the Earth in the laboratory. A Diamond Anvil Cell (DAC) uses two gem-quality diamonds with two smaller cutlet faces opposing each other. The difference in area between the table (large) and cutlet sides of the diamonds means that pressures exceeding 100 GPa can be achieved at the sample using hand tools to tighten the screws pushing the anvils together. A gasket, typically made from a strong metal such as rhenium, and with a small hole (perhaps 0.1 mm or smaller in diameter) to hold the sample, is used to contain the sample.
Because the force exerted by the diamonds is uni-axial, the pressure on the sample would be if the sample inside the gasket was in contact with the diamonds. To give a uniform (hydrostatic) pressure, the sample volume of the cells can be loaded with a noble gas or fluid such as helium or neon. With such a pressure medium, the diamonds press on the gaskets and the fluid to generate a uniform pressure more like is experienced by materials in the deep Earth.
Since diamonds are transparent to many kinds of light, the samples in a Diamond Anvil Cell can be heated with a laser that goes through the diamonds, with nearly the same path as the X-rays. At SEES beamlines, laser-heated Diamond Anvil Cells can reach pressures over 200 GPa and temperatures of 3000 K. A small ruby is typically included in the sample chamber so that the shift in Raman scattering from the ruby can be used to accurately measure the pressure.
X-ray diffraction measurements with a DAC are usually done with the X-rays traveling through the diamonds and the sample. The scattering patterns downstream of the sample are measured with an area detector camera.
Large Volume Press X-ray Diffraction
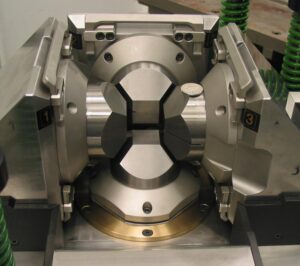
Large Volume Press Anvils
While the pressures in a diamond anvil cell can be very high, this requires the samples analyzed to be very small, typically 0.01 to 0.1 mm in size. That makes it challenging to get a complete thermodynamic understanding of the materials, to measure strain and deformation under load, or to perform other measurements, such as acoustic measurements or X-ray imaging on minerals under pressure. A Large Volume Press (LVP) uses much larger samples (cubic millimeters) and much larger anvils to generate pressures. Such presses can have a variety of configurations of their anvils, which are typically made from tungsten carbide and sometimes with tips made of sintered diamonds. Pressures up to 50 GPa can be achieved with such presses, and external heating can allow temperatures to 2500 K or so.
Large Volume Presses allow a variety of experiments to be done, including X-ray diffraction with either monochromatic or white X-ray beams. Such studies are often done to measure the complex relationship between Pressure, Volume, and Temperature — the equation of state — for minerals at conditions of the deep Earth. Because the samples are cubic millimeters in volume, X-ray imaging experiments such as X-ray Radiography and X-ray Tomography can be done on samples at high pressure and temperature. In addition, non-X-ray measurements such as acoustic emission can be made on mineral samples under extreme conditions, listening for faults and cracks in minerals that mimic earthquakes.
Inelastic X-ray Scattering
All of the scattering and diffraction measurements described above assume that X-rays are scattered or diffracted elastically, without losing any energy in the scattering process. Inelastic X-ray scattering happens when X-rays give some of their energy to the atoms or electrons in the sample. There are a few different phenomena that can cause inelastic X-ray scattering.